Antimatter propulsion has captured the collective imagination of scientists, engineers, and science fiction enthusiasts alike. It represents a potential revolution in space travel, allowing humanity to explore the cosmos at unprecedented speeds and efficiency. Despite its theoretical promise, antimatter-based propulsion systems remain a distant prospect, primarily due to the immense difficulties surrounding the production and storage of antimatter. This article provides an in-depth exploration of the current state of antimatter propulsion technology, the challenges faced, and potential future avenues for research and development.
1. Introduction
Humanity has long dreamt of traveling beyond our solar system. As we push the boundaries of space exploration, the quest for faster propulsion methods is becoming increasingly critical. Traditional rocket propulsion systems are limited by their reliance on chemical reactions, which impart substantial thrust but are inherently inefficient. Alternative methods, such as electric propulsion and solar sailing, while more efficient, typically provide insufficient force for rapid travel over long distances. This has led scientists to consider antimatter propulsion as a viable option for interstellar travel.
Antimatter, the mirror image of matter, consists of antiparticles that carry opposite charges to their corresponding particles in normal matter. When matter and antimatter come into contact, they annihilate each other in a release of energy described by Einstein's equation, E=mc2. This phenomenon presents a compelling opportunity for propulsion systems capable of generating massive amounts of energy from relatively small amounts of antimatter.
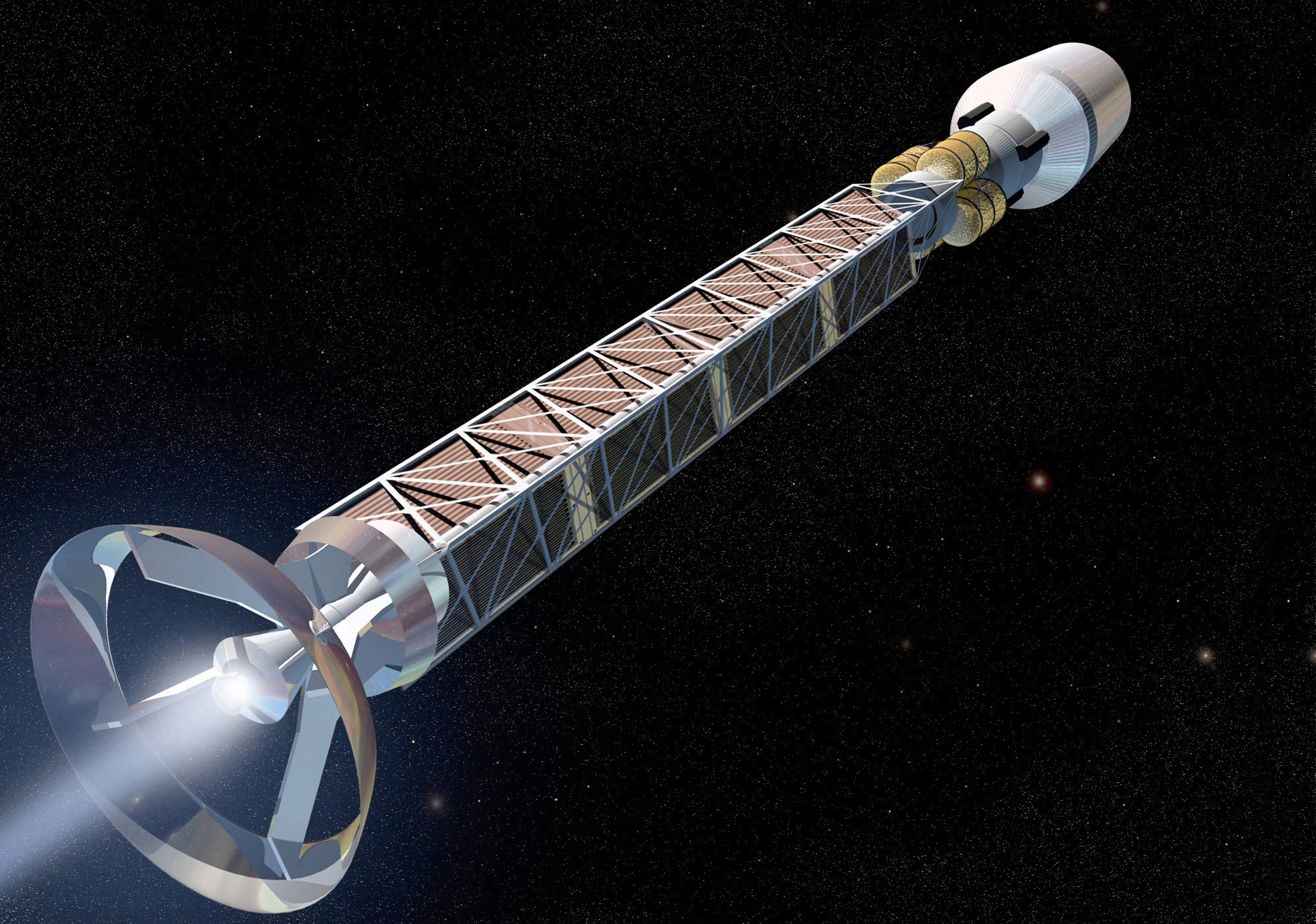
Artist's concept of an antimatter propulsion system. Credit: NASA/MFSC
2. The Science of Antimatter
The concept of antimatter was first proposed in the early 20th century, and the first antiparticle, the positron, was discovered by Carl Anderson in 1932. Antimatter is produced naturally in cosmic processes, such as in the interaction of cosmic rays with interstellar particles. However, producing antimatter in quantities sufficient for propulsion applications has proven extraordinarily difficult.
2.1 Antiparticles and Annihilation
Antiparticles correspond to each particle in standard matter. For example, the antiparticle of the electron (which carries a negative charge) is the positron (which carries a positive charge). The annihilation of a particle and its antiparticle releases energy, specifically in the form of gamma rays and other particles such as pions and kaons, which are produced at relativistic speeds. The energy yield from this annihilation reaction is staggering—one gram of antimatter can release about 1.8 × 1014 joules, a figure that is approximately eleven orders of magnitude higher than that of traditional rocket fuels.
2.2 Current Production Methods
Currently, the most effective method to produce antimatter involves proton collisions in particle accelerators. At the CERN laboratory, specifically the Antiproton Decelerator (AD), scientists can produce antiprotons by smashing protons together to create large amounts of energy, which can then result in the creation of antiparticles. However, the process is immensely energy-intensive and expensive—producing just ten nanograms of antiproton material costs over several million dollars.
Method | Energy Required | Production Rate |
---|---|---|
Particle Accelerators | 25 million kWh per gram | 10 nanograms/year |
Cosmic Ray Interactions | Variable | Natural production |
3. Challenges in Antimatter Propulsion
Despite the theoretical benefits of antimatter propulsion, several significant challenges hinder its practical implementation.
3.1 Storage Issues
One of the largest hurdles in antimatter propulsion is the storage of antimatter. Antimatter cannot come into contact with normal matter, as even minor contact would result in annihilation, releasing energy and losing the antimatter intended for propulsion. Therefore, antimatter must be stored in vacuum chambers using electromagnetic traps, a method that has been successfully demonstrated only for short periods (up to 16 minutes at CERN).
3.2 Economic Feasibility
The production of antimatter is prohibitively expensive. Estimates suggest that producing one gram of antimatter could cost upwards of $4 million—a price tag that is untenable for current space funding models.
3.3 Scalability
Even if production and storage challenges are addressed, scaling up production to a level suitable for a crewed spacecraft mission to another star requires advances not only in technology but also in funding and international collaboration. The sheer amount of antimatter required for significant propulsion—likely grams to kilograms—far exceeds current production capabilities.
3.4 Long-Term Effects of Exposure
Potential long-term exposure to antimatter for both crew and equipment poses additional unknown risks. Research is still ongoing to fully understand the effects of this unique energy source on human physiology, spacecraft materials, and the broader cosmic environment.
4. Current Research and Development
Several research groups and institutions are exploring viable technologies that may facilitate antimatter propagation, focusing on production methods, storage solutions, and potential applications.
4.1 Advancements in Particle Physics
Researchers at CERN and other leading institutions are continuously looking at ways to enhance the efficiency of antimatter production. New techniques or improvements to existing particle acceleration methods could significantly increase the yield of antimatter, making it a more accessible energy source.
4.2 Innovative Storage Solutions
Efforts are underway to design innovative magnetic or electromagnetic fields capable of trapping antimatter for extended periods. These advancements could enable the safe handling and utilization of antimatter, paving the way for practical propulsion applications.
4.3 Alternative Energy Solutions
Some scientists propose that developments in fusion energy could provide cheaper energy sources for antimatter production. If researchers can make progress in sustainable fusion technology, they may be able to mitigate the costs currently associated with antimatter production.
5. Future Directions
While the prospect of antimatter propulsion remains far from realization, there are hopeful signs that progress is being made. Future directions for research in antimatter propulsion may include the following:
- Development of Efficient Antimatter Production Techniques: Streamlining production methods could reduce costs and increase availability.
- Advancements in Storage Technologies: Innovations in electromagnetic trapping could allow for longer retention periods.
- Exploration of new propulsion systems: Hybrid systems utilizing antimatter in combination with conventional methods might be explored.
- International Collaboration: Given the substantial resources required, global cooperation among nations and space agencies could expedite antimatter research.
- Research on Safety Protocols: Developing safety protocols for antimatter usage is crucial to ensure safe practices on missions that use this technology.
6. Conclusion
Antimatter propulsion has the potential to revolutionize space travel and open new avenues for exploration in our universe. However, substantial technical, economic, and safety challenges must be addressed before it can become a reality. Continued research and technological advancements may one day allow humanity to harness the incredible power of antimatter, propelling us toward the stars.
For More Information
- Future of Antimatter Production, Storage, Control, and Annihilation Applications in Propulsion Technologies
- It’s Official, Antimatter Falls Down in Gravity, Not Up
- Are There Antimatter Galaxies?
- Spectrum of Antimatter Observed for First Time
References: All content utilized for this article is sourced from Universe Today.